Pseudomonas syringae
What is Pseudomonas syringae?
The bacteria Pseudomonas syringae is a common plant pathogen mostly found in stems and leaves [1]. It infects a wide range of plants—including fruit trees, greenhouse vegetables, ornamentals, and field crops. Annually, the bacteria cause billions of dollars of damage worldwide from lost fruits, damaged trees, unmarketable ornamentals, and more. In 2010, farmers in New Zealand experienced a loss of $450 million worth of kiwi [2–7]. As an opportunistic pathogen, the bacteria can only infect a plant via openings like the stomata and wounds. Once inside the host, it employs various ways to attack the plant with virulence factors [1]. The most reported methods are releasing plant toxins and producing a protein that encourages ice formation in the plant [1,2,8]. The P. syringae species complex comprises over 50 pathovars, each infecting a specific group of plants [7,9,10].
Virulence factors – molecules that help a pathogen invade and cause disease in a host
Pathovars – strains of an organism that can cause infections and disease in a host
Table 1. A short list of Pseudomonas syringae pathovars and their target plant examples.*
Species |
Pathovar |
Target Plant |
Pseudomonas syringae |
pv. aceris |
maple |
Pseudomonas syringae |
pv. actinidiae |
kiwifruit |
Pseudomonas syringae |
pv. aesculi |
horse chestnut |
Pseudomonas syringae |
pv. alisalensis |
Broccoli, cabbage |
Pseudomonas syringae |
pv. aptata |
beets |
Pseudomonas syringae |
pv. atrofaciens |
wheat |
Pseudomonas syringae |
pv. cannabina |
cannabis |
Pseudomonas syringae |
pv. coryli |
European hazelnut |
Pseudomonas syringae |
pv. dysoxylis |
kohekohe tree |
Pseudomonas syringae |
pv. glycinea |
soybean |
Pseudomonas syringae |
pv. japonica |
barley |
Pseudomonas syringae |
pv. lachrymans |
cucurbits |
Pseudomonas syringae |
pv. lapsa |
wheat |
Pseudomonas syringae |
pv. maculicola |
collards, turnip, mustard, rape salad greens, cauliflower |
Pseudomonas syringae |
pv. mori |
cannabis |
Pseudomonas syringae |
pv. morsprunorum |
stone fruits |
Pseudomonas syringae |
pv. panici |
panicum grass |
Pseudomonas syringae |
pv. papulans |
apples |
Pseudomonas syringae |
pv. persicae |
nectarine, peach |
Pseudomonas syringae |
pv. phaseolicola |
beans, lettuce |
Pseudomonas syringae |
pv. pisi |
peas |
Pseudomonas syringae |
pv. solidagae |
raspberry, blackberry |
Pseudomonas syringae |
pv. syringae |
lilac, plum, Phaseolus beans, mango, apple, pear, stone fruits, pepper, cucurbits, most ornamental flowers |
Pseudomonas syringae |
pv. tomato |
tomato, pepper |
*Information summarized from [11]. Additional information added to this table are from [11–23].
Historically, P. syringae is known as the model plant pathogen; studying the bacteria created many advancements in agriculture and plant protection [1]. However, the study of bacteria is shifting from disease to ecology. Recent studies show that P. syringae is not just a plant pathogen but a bacterium that lives and influences the Earth’s water cycle [24]. Therefore, to understand how to control the bacteria in an agricultural setting, we must understand that it is a part of all naturally occurring bodies of water and the Earth’s water cycle.
Symptoms of Pseudomonas syringae
Symptoms of P. syringae depend on the host, with most symptoms appearing above the ground: stems, leaves, flowers, and fruit. Characteristic symptoms can include the following [2,11,12,16,17]:
- Stem and trunk cankers with a gummy substance are often found coming from the open wound
- Blackened twig (shoot-tip dieback)
- Discoloured or blackened leaves
- Bacterial leaf blight
- Peppery leaf spots
- Necrotic leaf spots
- Brown/blackened flowers (blossom blast)
- Dead, dormant buds
- Bacterial canker on fruits
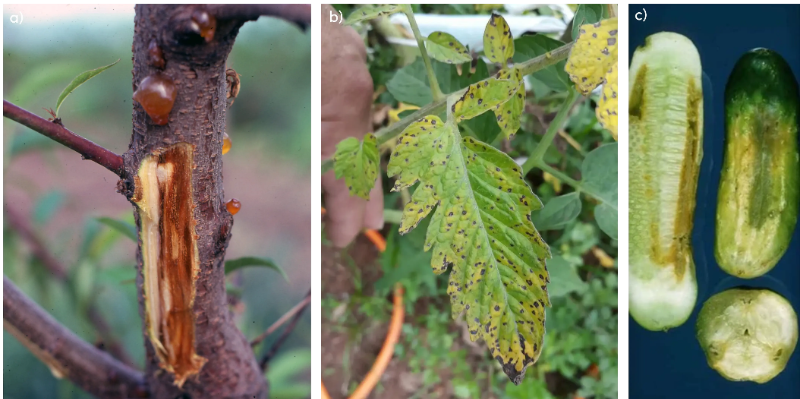
We provide great overviews of many agricultural microorganisms. Subscribe to stay updated!
Life Cycle and Infection Stages
Before understanding the life cycle and the infection stages of P. syringae, it is essential to remember that the disease only occurs after P. syringae enters the plant. There are two phases of the life cycle:
1. Epiphytic Phase
The bacteria live outside the plant, typically on the surface of stems, leaves, flowers, fruits, and other above-ground plant parts. It lives in balance with other microorganisms on the plant’s surface [1,25]. As a result, the bacteria can complete its lifecycle and multiply. The bacteria can then transfer to other plants via water, insects, or other mechanical modes of transportation, such as agricultural tools [26,27].
Interestingly, P. syringae can produce ice nucleation proteins that help water crystalize and penetrate the plant’s surface forming wounds [25,28]. Note that not all pathovars of P. syringae can induce ice crystals to form. While P. syringae pv. syringae have been found to have the ice-forming ability, P. syringae pv. tomato does not [25].
2. Endophytic Phase
The endophytic phase is the disease state. First, P. syringae enters the plant through the stomata or open wounds. It can produce a compound called coronatine that tricks the stomata into opening [29]. Next, it grows and multiplies in the space between plant cells called the apoplast. Finally, to protect itself from the plant’s defences, it uses T3SS effectors (T3Es) and other virulence factors to interfere with the plant’s immune system [1,25,30]. As the bacterial population multiplies, it grows beyond the capabilities of the plant’s local immune system. the infected area will show visual symptoms, and the plant will start to die. The bacteria can also spread inside the plant through the plant’s vascular system [31].
apoplast – the space between cells where water and materials can freely flow, including the cell wall
T3SS effectors (T3Es) – needle-like compounds produced by bacteria that secrete molecules that interfere with the plant’s immune system
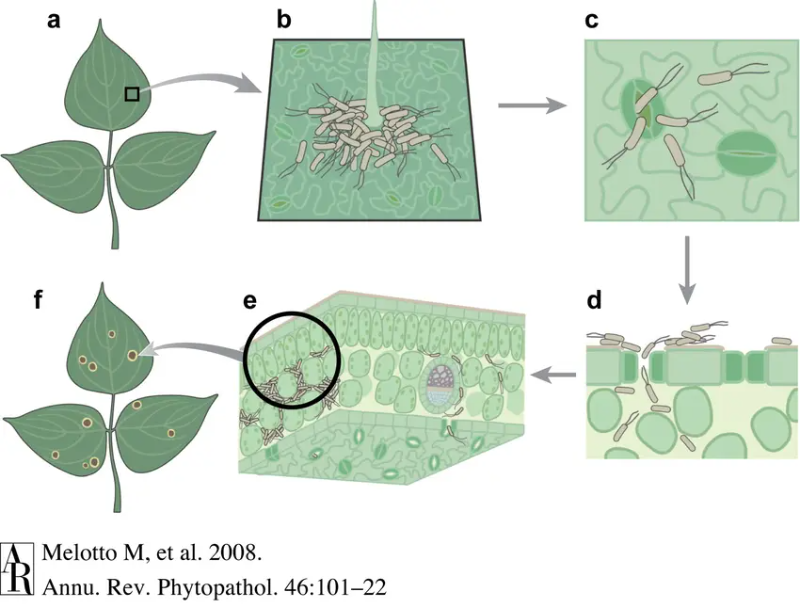
Growth conditions for Pseudomonas syringae
P. syringae is a ubiquitous bacterium that grows in many different conditions. The disease phase of the bacteria favours wet and humid conditions because it helps with the following[1,32,33]:
- penetration into a plant by keeping the stomata open
- quicker rate of infection due to the increased reproduction rate in the apoplast
- movement of the bacteria to different plants
It favours cooler temperatures, with bacteria preferring 18 to 24°C [34]. Furthermore, it also can grow between 0°C to 4°C [35,36]. For outdoor plants susceptible to frost, P. syringae can invade and provoke a disease phase during wet and cooler times of the year, especially in spring [37].
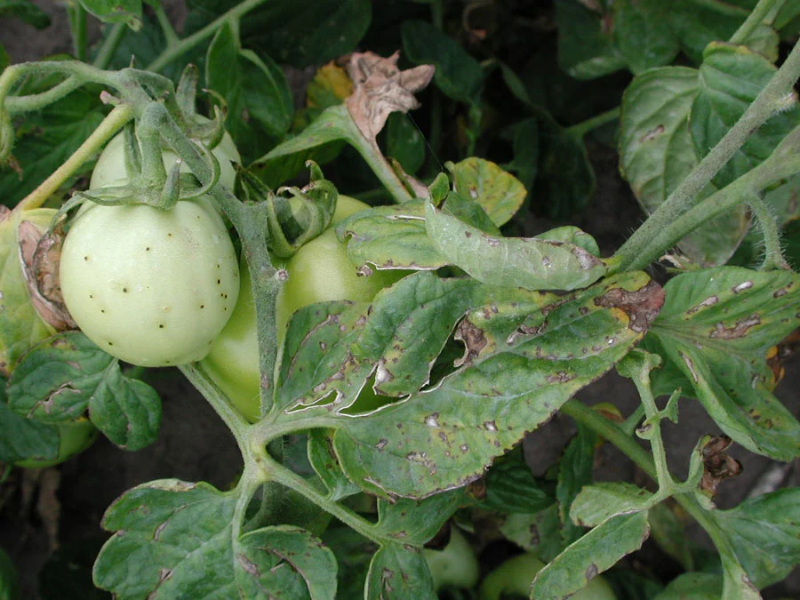
Methods of Prevention and Control
Best Practices against Pseudomonas syringae
P. syringae spreads through water, insects, and contaminated surfaces. The bacteria is found everywhere with naturally occurring water, and it is a common bacteria found in the natural environment. Therefore, prevention is the best way to control it. Here are a couple of ways that growers can decrease the risk of infection [2,3,23,37–40]
- Use new containers or properly sanitize previously used containers
- Minimize contact with soil outside the growing areas or untreated water from the outside water sources (rain, wind, pond water, etc.)
- Prune infected plants to prevent the spread of the bacteria to the rest of the crop
- Avoid hose ends and other equipment from touching the ground or soil
- Avoid using contaminated equipment with cuttings
- Water your crops in the morning so they can dry during warmer times of the day
- Increase the airflow between plant leaves
- Increase water drainage
- Be diligent about IPM management, as insects can also be a source of contamination
- Be vigilant and diagnose quickly to allow time to apply control strategies
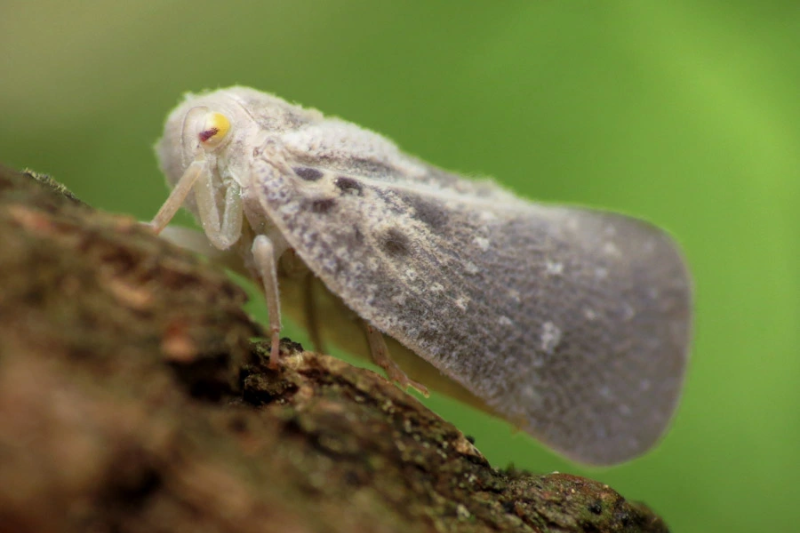
Resistant Varieties
Plant varieties resistant or less susceptible to P. syringae infections are commercially available. For example, lilac varieties Syringae josikaea, S. komarowii, S. microphylla, S. pekinensis, and S. reflexa are less susceptible than the common lilac cultivar [2]. For tomatoes, Ontario 7710 breeding line has resistance to P. syringae pv tomato, which is reported to be a source of resistance for other cultivars [41, 42]. Some commercially available cucumber varieties have intermediate resistance to Pseudomonas syringae pv. lachrymans [43].
Treatments for Pseudomonas syringae
A preventative method that growers should consider are biocontrols that actively work against pathogens. Currently, only Bacillus subtilis and rhizobacteria are commercially available [44]. Traditional chemical treatments are available for treating infections. Typical commercially available controls are either copper-based or bactericides [2, 40, 44]. Please always read the labels and note that different treatments exist for plant types and geographical regions. In some plants, spraying during the fall helps decrease bacterial populations and prepares the plant for when it is more vulnerable in spring. However, it is essential to note that copper treatments can damage young plants and that some P. syringae pathovars are resistant to copper [2].
Conclusion
Pseudomonas syringae is an opportunistic pathogen that can be irritating to control once the disease phase starts. The symptoms range from treatable to systemic disease. Therefore, preventative practices are the most effective way of keeping it at bay. learn more about how early detection can help, feel free to email us at [email protected].
Disclaimer:
The information we present in Pathogen Profile is based on collating published peer-reviewed scientific literature and sources we think are reliable. This is by no means an exhaustive review of pathogens. Pathogen Profile gives a small glimpse of what is known about pathogens. We encourage growers to do more research on the pathogens concerning their crops and hydroponic systems. We are not plant pathologists; thus, the information presented in the Pathogen Profile should not be used as professional advice to treat pathogens or operate your hydroponic system.
References
- Xin, X.-F., Kvitko, B., & He, S. Y. (2018). Pseudomonas syringae: what it takes to be a pathogen. Nature Reviews Microbiology, 16(5), 316–328. https://doi.org/10.1038/nrmicro.2018.17
- Moorman, G. W. (2014). Pseudomonas syringae: A common pathogen on woody plants. Pennsylvania State University Extension. Retrieved from https://extension.psu.edu/pseudomonas-syringae-a-common-pathogen-on-woody-plants
- Moore, L. W., & Pscheidt, J. W. (n.d.). Diseases Caused by Pseudomonas syringe. Pacific Norhwest Pest Management Handbooks. Retrieved from https://pnwhandbooks.org/plantdisease/pathogen-articles/pathogens-common-many-plants/bacteria-other-prokaryotes/diseases
- The Figi Times. (2017). $1.2b loss. The Fiji Times. Retrieved from https://www.fijitimes.com/1-2b-loss/
- Mandow, N. (2021). The case of the catastrophic bacteria and Govt’s liability. Newsroom. Retrieved from https://www.newsroom.co.nz/must-govt-pay-well-see
- Wight, A. (2020). This Colombian Biologist Is Protecting The Future Of Sweet potato. Forbes. Retrieved from https://www.forbes.com/sites/andrewwight/2020/03/12/this-colombian-biologist-is-protecting-the-future-of-sweetpotato/?sh=530b0a7971c3
- Chen, H., Chen, J., Zhao, Y., Liu, F., & Fu, Z. Q. (2022). Pseudomonas syringae pathovars. Trends in Microbiology, 30(9), 912–913. https://doi.org/https://doi.org/10.1016/j.tim.2022.03.002
- de Araujo, G. G., Rodrigues, F., Gonçalves, F. L. T., & Galante, D. (2019). Survival and ice nucleation activity of Pseudomonas syringae strains exposed to simulated high-altitude atmospheric conditions. Scientific Reports, 9(1), 7768. https://doi.org/10.1038/s41598-019-44283-3
- Bull, C. T., De Boer, S. H., Denny, T. P., Firrao, G., Saux, M. F.-L., Saddler, G. S., … Takikawa, Y. (2010). COMPREHENSIVE LIST OF NAMES OF PLANT PATHOGENIC BACTERIA, 1980-2007. Journal of Plant Pathology, 92(3), 551–592. Retrieved from http://www.jstor.org/stable/41998846
- Gomila, M., Busquets, A., Mulet, M., García-Valdés, E., & Lalucat, J. (2017). Clarification of Taxonomic Status within the Pseudomonas syringae Species Group Based on a Phylogenomic Analysis . Frontiers in Microbiology . Retrieved from https://www.frontiersin.org/articles/10.3389/fmicb.2017.02422
- Pseudomonas syringae. (2022). Wikipedia. Retrieved from https://en.wikipedia.org/wiki/Pseudomonas_syringae
- Bestwick, C. S., Bennett, M. H., & Mansfield, J. W. (1995). Hrp Mutant of Pseudomonas syringae pv phaseolicola Induces Cell Wall Alterations but Not Membrane Damage Leading to the Hypersensitive Reaction in Lettuce. Plant Physiology, 108(2), 503–516. Retrieved from http://www.jstor.org/stable/4276573
- Kennelly, M. M., Cazorla, F. M., de Vicente, A., Ramos, C., & Sundin, G. W. (2007). Pseudomonas syringae Diseases of Fruit Trees: Progress Toward Understanding and Control. Plant Disease, 91(1), 4–17. https://doi.org/10.1094/PD-91-0004
- Chin, A., & Miller, S. A. (n.d.). Peppery Leaf Spot. Ohio State University: VEGETABLE DISEASE FACTS. Retrieved from https://u.osu.edu/vegetablediseasefacts/brassica-diseases/peppery-leaf-spot/
- Wechter, W. P., Keinath, A. P., Farnham, M. W., & Smith, J. P. (2009). First Report of Bacterial Leaf Blight on Broccoli and Cabbage Caused by Pseudomonas syringae pv. alisalensis in South Carolina. Plant Disease, 94(1), 132. https://doi.org/10.1094/PDIS-94-1-0132C
- Ivanović, M., Prokić, A., Gašić, K., Menković, J., Kuzmanovic, N., Zlatkovic, N., & Obradoviċ, A. (2022). Characterization of Pseudomonas syringae strains associated with shoot blight of raspberry and blackberry in Serbia. Plant Disease. https://doi.org/10.1094/PDIS-06-22-1425-RE
- 瀧川雄一, 露無慎二, & 後藤正夫. (1991). Pseudomonas syringae pv. acerisによるトウカエデ斑点細菌病(新称)について. 日本植物病理学会報, 57(5), 724–728. https://doi.org/10.3186/jjphytopath.57.724
- Gutiérrez-Barranquero, J. A., Cazorla, F. M., & de Vicente, A. (2019). Pseudomonas syringae pv. syringae Associated With Mango Trees, a Particular Pathogen Within the “Hodgepodge” of the Pseudomonas syringae Complex. Frontiers in Plant Science. Retrieved from https://www.frontiersin.org/articles/10.3389/fpls.2019.00570
- Sharon, E., Okon, Y., Bashan, Y., & Henis, Y. (1982). Detached leaf enrichment: a method for detecting small numbers of Pseudomonas syringae pv. tomato and Xanthomonas campestris pv. vesicatoria in seed and symptomless leaves of tomato and pepper. Journal of Applied Bacteriology, 53(3), 371–377. https://doi.org/https://doi.org/10.1111/j.1365-2672.1982.tb01285.x
- BUONAURIO, R., & SCORTICHINI, M. (1994). Pseudomonas syringae pv. syringae on pepper seedlings in Italy. Plant Pathology, 43(1), 216–219. https://doi.org/https://doi.org/10.1111/j.1365-3059.1994.tb00573.x
- Słomnicka, R., Olczak-Woltman, H., Bartoszewski, G., & Niemirowicz-Szczytt, K. (2015). Genetic and pathogenic diversity of Pseudomonas syringae strains isolated from cucurbits. European Journal of Plant Pathology, 141(1), 1–14. https://doi.org/10.1007/s10658-014-0524-4
- McPartland, J. M. (1996). A review of Cannabis diseases. Journal of the International Hemp Association, 3(1), 19–23. Retrieved from https://druglibrary.org/olsen/hemp/iha/iha03111.html
- UMass Center for Agriculture, Food, and the E. (2022). Geranium – Bacterial leaf spot (Pseudomonas syringae). UMass Extension Greenhouse Crops and Floriculture Program. Retrieved from https://ag.umass.edu/greenhouse-floriculture/photos/geranium-bacterial-leaf-spot-pseudomonas-syringae
- Morris, C. E., Monteil, C. L., & Berge, O. (2013). The Life History of Pseudomonas syringae: Linking Agriculture to Earth System Processes. Annual Review of Phytopathology, 51(1), 85–104. https://doi.org/10.1146/annurev-phyto-082712-102402
- S., H. S., & D., U. C. (2000). Bacteria in the Leaf Ecosystem with Emphasis on Pseudomonas syringae—a Pathogen, Ice Nucleus, and Epiphyte. Microbiology and Molecular Biology Reviews, 64(3), 624–653. https://doi.org/10.1128/MMBR.64.3.624-653.2000
- Morris, C. E., Sands, D. C., Vinatzer, B. A., Glaux, C., Guilbaud, C., Buffière, A., … Thompson, B. M. (2008). The life history of the plant pathogen Pseudomonas syringae is linked to the water cycle. The ISME Journal, 2(3), 321–334. https://doi.org/10.1038/ismej.2007.113
- Melotto, M., Underwood, W., & He, S. (2008). Role of Stomata in Plant Innate Immunity and Foliar Bacterial Diseases. Annual review of phytopathology, 46, 101–122. https://doi.org/10.1146/annurev.phyto.121107.104959
- Lindow, S. E., Hirano, S. S., Barchet, W. R., Arny, D. C., & Upper, C. D. (1982). Relationship between Ice Nucleation Frequency of Bacteria and Frost Injury. Plant Physiology, 70(4), 1090–1093. Retrieved from http://www.jstor.org/stable/4267645
- Katsir, L., Schilmiller, A. L., Staswick, P. E., He, S. Y., & Howe, G. A. (2008). COI1 is a critical component of a receptor for jasmonate and the bacterial virulence factor coronatine. Proceedings of the National Academy of Sciences, 105(19), 7100–7105. https://doi.org/10.1073/pnas.0802332105
- Zheng, X., Spivey, N. W., Zeng, W., Liu, P.-P., Fu, Z. Q., Klessig, D. F., … Dong, X. (2012). Coronatine Promotes <em>Pseudomonas syringae</em> Virulence in Plants by Activating a Signaling Cascade that Inhibits Salicylic Acid Accumulation. Cell Host & Microbe, 11(6), 587–596. https://doi.org/10.1016/j.chom.2012.04.014
- Misas-Villamil, J. C., Kolodziejek, I., & van der Hoorn, R. A. L. (2011). Pseudomonas syringae colonizes distant tissues in Nicotiana benthamiana through xylem vessels. The Plant Journal, 67(5), 774–782. https://doi.org/https://doi.org/10.1111/j.1365-313X.2011.04632.x
- Xin, X.-F., Nomura, K., Aung, K., Velásquez, A. C., Yao, J., Boutrot, F., … He, S. Y. (2016). Bacteria establish an aqueous living space in plants crucial for virulence. Nature, 539(7630), 524–529. https://doi.org/10.1038/nature20166
- Panchal, S., Chitrakar, R., Thompson, B. K., Obulareddy, N., Roy, D., Hambright, W. S., & Melotto, M. (2016). Regulation of stomatal defense by air relative humidity. Plant physiology, 172(3), 2021–2032. https://doi.org/10.1104/pp.16.00696
- OMAFRA. (n.d.). BACTERIAL SPECK. Ontario CropIPM. Retrieved from http://www.omafra.gov.on.ca/IPM/english/tomatoes/diseases-and-disorders/bacterial-speck.html
- Seshu, K. G., V., J. M., & K., R. M. (2002). Low-Temperature-Induced Changes in Composition and Fluidity of Lipopolysaccharides in the Antarctic Psychrotrophic Bacterium Pseudomonas syringae. Journal of Bacteriology, 184(23), 6746–6749. https://doi.org/10.1128/JB.184.23.6746-6749.2002
- Shivaji, S., Rao, N. S., Saisree, L., Sheth, V., Reddy, G. S., & Bhargava, P. M. (1989). Isolation and identification of Pseudomonas spp. from Schirmacher Oasis, Antarctica. Applied and Environmental Microbiology, 55(3), 767–770. https://doi.org/10.1128/aem.55.3.767-770.1989
- University of California IPM. (2017). Bacterial blast, blight, and canker—Pseudomonas syringae. How to Manage Pests Pests in Gardens and Landscapes. Retrieved from http://ipm.ucanr.edu/PMG/GARDEN/PLANTS/DISEASES/baclfblight.html
- University of California IPM. (2017). Bacterial Leafspot. How to Manage Pests UC Pest Management Guidelines. Retrieved from http://ipm.ucanr.edu/PMG/r104100311.html
- Government of Canada. (2015). Bacterial Blight: Pseudomonas syringae pathovar (pv.) Syringae. Diseases and pests in agroforestry. Retrieved from https://agriculture.canada.ca/en/agricultural-production/agricultural-pest-management/diseases-and-pests-agroforestry/bacterial-blight
- UMass Extension Greenhouse Crops & Floriculture Program. (2022). Impatiens – Leaf spot (Pseudomonas syringae). UMass Extension Greenhouse Crops & Floriculture Program. Retrieved from https://ag.umass.edu/greenhouse-floriculture/photos/impatiens-leaf-spot-pseudomnas-syringae
- Kerr, E. A., & Cook Simcoe, Ont. (Canada)), F. I. (Hort. E. S. (1983). Ontario 7710 – a tomato breeding line with resistance to bacterial speck, Pseudomonas syringae pv. tomato (Okabe). (F. A. O. of the UN, Ed.).
- EUROPEAN SEED ASSOCIATION. (2014). EUROPEAN SEED ASSOCIATION – CODE FOR RESISTANCE HARMONIZATION. Retrieved from https://www.medhermes.net/public/prodotti/esa-hrt-tomato 12052014.pdf
- Growing Produce. (2022). The Latest Cucumber Varieties You Need to Know. Retrieved from https://www.growingproduce.com/vegetables/11-of-the-latest-cucumber-varieties-you-need-to-know/#slide=152800-136706-5
- Bashan, Y. (1997). Alternative Strategies for Controlling Plant Diseases Caused by Pseudomonas syringae BT – Pseudomonas Syringae Pathovars and Related Pathogens. In K. Rudolph, T. J. Burr, J. W. Mansfield, D. Stead, A. Vivian, & J. von Kietzell (Eds.), (pp. 575–583). Dordrecht: Springer Netherlands. https://doi.org/10.1007/978-94-011-5472-7_105
David Santos is a Biotech and Agriscience Advisor at Healthy Hydroponics